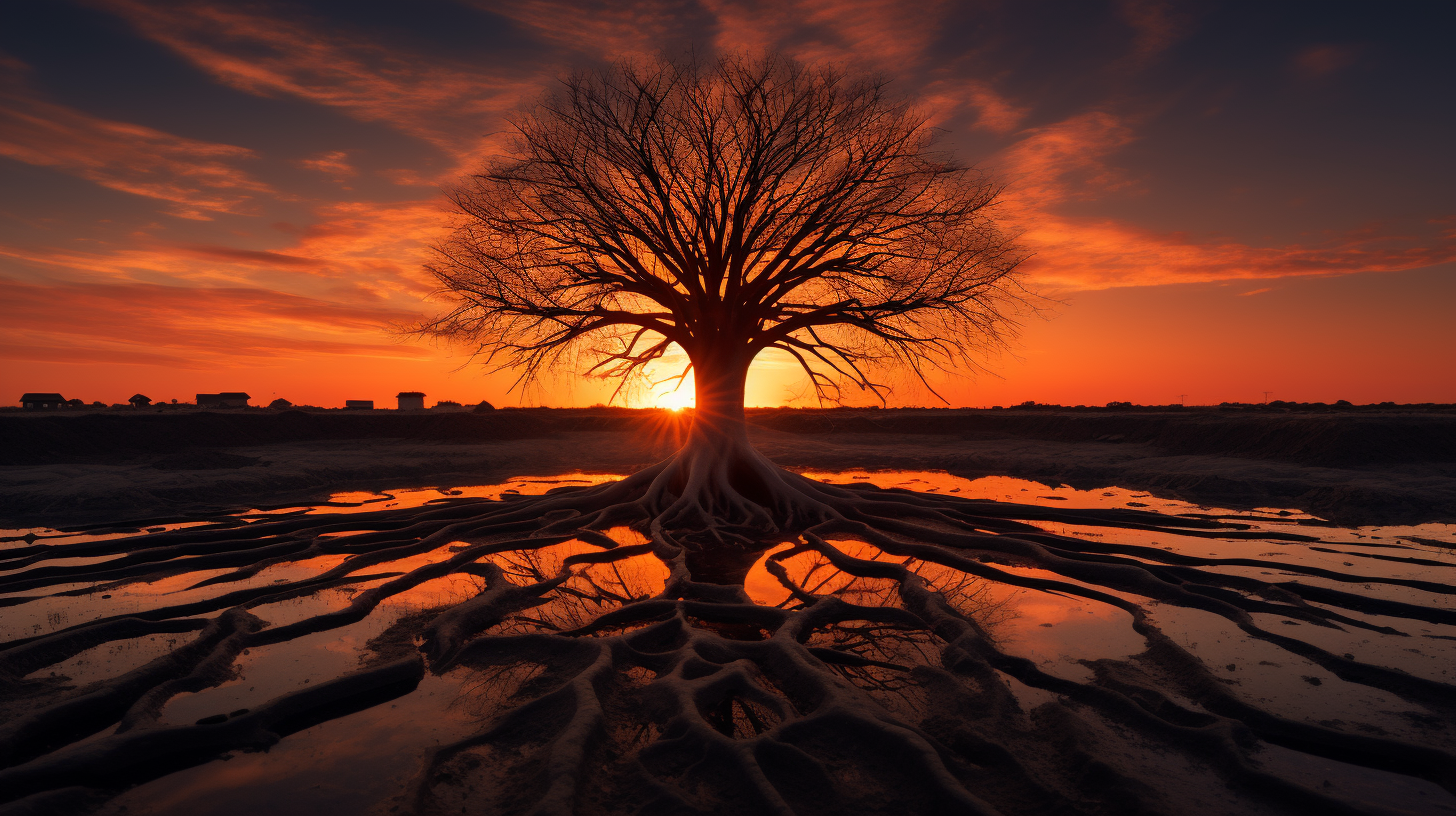
Master the art of allocating reliability and quality goals to the different parts of your system to slash costs, speed up schedules, and embed accountability.
Reliability and Quality Allocation Course
(Virtual or Onsite – 24 hours)
$2040.00 USD
Discount opportunities available for teams & groups.
Contact us for more information on securing a discounted rate.
Course Curriculum
Course Overview
Become an asset to your organization by creating meaningful reliability (design) and quality (manufacturing) targets for the different parts of your product. Getting these targets right means giving the most ‘challenging’ parts the ‘easiest’ targets, and the ‘simplest’ parts the ‘hardest’ targets. Why? This dramatically speeds up your production and slashes costs, and speeds everything up as your entire project stops revolving around one or two ‘critical’ parts that can never achieve their allocated targets, while other parts can more easily and cheaply have their performance improved to meet system requirements. Reliability and quality allocation is often essential for large, complex projects where different teams and organizations must independently (and sometimes in other countries) achieve their own targets to meet system requirements. Students will learn how to easily develop meaningful and useful targets for their system's different parts that allow them to continually monitor and achieve reliability and quality targets.

Course Objectives
Students who complete this course will be able to:
· confidently manage their reliability and quality ‘budgets’ by knowing what each component or part of a system or product needs to achieve and quickly working out which ones will need more help.
· reduce operational costs by prioritizing the areas of your product or system that need to be the focus of continual improvement (and not wasting time and money improving the performance of components and parts that don’t drive poor reliability and quality.
· know if and when you need redundancy in your product to ensure that you don’t unnecessarily include additional components that will increase the cost, size, and mass.
· support systems engineering frameworks that allocate reliability and quality targets to different components and subsystems designed and created by other groups and teams under different contracts.
· make data-driven decision-making for reliability and quality strategies that provide confidence and eliminate stress.
· use a quality and reliability mindset that results in robust, reliable, and quality products that enhance customer satisfaction and loyalty as their products are the ‘most like.’
· foster a culture of continual improvement where different design teams have tailored and feasible reliability and quality goals and targets they are motivated to achieve.
· create accountability where different teams and groups have their own, clearly defined targets and goals that are not contingent on anyone else.
· proactively risk manage and mitigate (including safety & compliance) that forces a systematic approach to identifying potential failure modes and their consequences across the organization.
Course Structure
The course comprises 24 1-hour lessons that can be delivered virtually or onsite.
Course Materials
-
For students attending the virtual course, 12 months of access to recorded lessons.
-
Accompanying Reliability and Quality Allocation guidebook that contains all the content of the course
-
Ongoing support in the form of questions and answers.
Assessment Methods
-
· There are a total of eight (8) worked exercises throughout the course will be used to assess students’ competency.
-
A certificate will be issued to each student who successfully completes the course.
Course Duration
This is a 24-hour course that can be delivered onsite or virtually over 3 days (8 hours of lessons per day) or 4 days (6 hours of lessons per day).
Enrollment Requirements
This course is designed for professionals overseeing or assisting with designing products and systems, or those responsible for establishing quality goals for manufacturing and mass production. No prior reliability, quality, probability, or statistics knowledge is required.
Course Outline (1-hour lesson descriptions)
-
Lesson 1: Introduction to Fault Tree Analysis (FTA)This lesson establishes the foundation of Fault Tree Analysis, outlining its core applications in system reliability, root cause analysis (RCA), and proactive design improvements. Learners explore key concepts like "top events" and fault tree logic, setting the stage for data-driven decision-making in risk assessment.
-
Lesson 2: Understanding System ReliabilityReliability is both a measurable and predictable aspect of system performance. This lesson covers key failure metrics, warranty considerations, and maintenance strategies, showing how FTA helps model and enhance system durability and dependability. Lesson 2 introduces system reliability analysis, focusing on how the reliability of individual components determines overall system performance. You'll learn how failure is a random but predictable process, explore key reliability metrics, and understand how factors like warranty periods and maintenance strategies impact product success. The lesson also covers fault tree modelling of system reliability, illustrating how things like series and parallel system structures influence failure risk and redundancy.
-
Lesson 3: Modeling Complex SystemsComplex systems require logical breakdowns into fault trees. This lesson explores series and parallel system structures, real-world case studies (pumping stations, nuclear plants), and logic gates (AND, OR) to analyze failure propagation. Lesson 3 focuses on modeling the reliability of increasingly complex systems using fault trees. It introduces methods for identifying overarching series or parallel structures within a system and progressively breaking them down into smaller subsystems until all component failures are represented as basic events. The lesson applies this process to real-world examples, including a pumping station and a nuclear power plant’s secondary cooling loop, demonstrating how to use logical structures like ‘AND’ and ‘OR’ gates. Additionally, it explores alternative ways to represent logic gates and illustrates how faults propagate through a system, leading to potential failures.
-
Lesson 4: More Fault Tree FunctionalityFTA isn’t just about component failure—it also considers human error, environmental factors, and system interactions. Learners explore undeveloped events, link events, and success trees, broadening their analytical approach. Lesson 4 expands FTA beyond basic component failures, incorporating additional events such as human errors and environmental effects. It introduces key concepts like undeveloped events, which represent incomplete or unavailable system information, and link events, which reference existing fault trees to reduce duplication. The lesson also explores intermediate events for better system understanding and introduces 'k out of n' gates for load-sharing systems. Finally, it contrasts fault trees with success trees, which model system functionality rather than failure, offering an alternative perspective on reliability analysis.
-
Lesson 5: Series and Parallel Systems ReliabilityLesson 5 covers series and parallel systems reliability analysis where simple equations can be used to convert component reliability characteristics into system reliability characteristics. Series systems fail when any component fails, making the least reliable component the main driver of system reliability. Parallel systems, in contrast, continue functioning as long as at least one component works, making them more reliable overall. The lesson explores how variations in component reliability affect overall system performance.
-
Lesson 6: Series & Parallel Systems (Exercises)Lesson 6 focuses on applying reliability equations to analyze series and parallel system configurations. Series systems fail when any component fails, while parallel systems fail only when all components fail. The lesson walks through practical examples, demonstrating how redundancy in parallel systems enhances reliability, while adding components to series systems reduces overall reliability. Engineers must balance these trade-offs while designing reliable systems and interpreting reliability curves over time.
-
Lesson 7: Analyzing 'K' out of 'N' SystemsA ‘𝑘’ out of ‘𝑛’ system is a redundancy-based configuration where at least ‘𝑘’ out of ‘𝑛’ components must function for the system to remain operational. These systems are distinct from purely series or parallel systems and exhibit unique reliability characteristics, often balancing the early failure risks of series systems and the extended functionality of parallel systems. Their design can improve system reliability, reduce physical size, and enhance maintainability while providing partial functionality even in a failed state. Understanding their behaviour, including reliability equations and failure probabilities, is crucial for optimizing system performance and redundancy strategies.
-
Lesson 8: Analyzing 'K' out of 'N' Systems Worked ExercisesLesson 8 focuses on applying reliability equations to analyze ‘𝑘’ out of ‘𝑛’ systems that require at least ‘𝑘’ components to function for the system to be operational. These systems improve reliability, maintainability, and availability by enabling repairs while the system remains operational. Their reliability can be analyzed using factorial-based equations, helping engineers determine their effectiveness over time. Understanding these systems is crucial for optimizing system design, balancing redundancy with efficiency, and ensuring high availability in critical applications.
-
Lesson 9: Analyzing ‘K’ out of ‘N’ Systems with Different ComponentsPrevious lessons focusing on ‘𝑘’ out of ‘𝑛’ system reliability are based on systems with identical components. Lesson 9 explores the reliability analysis of ‘𝑘’ out of ‘𝑛’ systems with different components, highlighting how varying component reliability characteristics impact overall system performance. It introduces event trees as a tool for modeling ‘𝑘’ out of ‘𝑛’ system reliability where components are different. The lesson illustrates how system reliability can align with the ‘𝑘th most reliable’ component and how event trees help visualize potential failure pathways. By applying these concepts to real-world scenarios, such as nuclear safety systems and redundant pump configurations, the lesson emphasizes the importance of understanding sequential failures and probabilistic outcomes in complex systems.
-
Lesson 10: Using Reliability CurvesLesson 10 explores the use of reliability curves in system design, focusing on how different configurations impact overall reliability and warranty periods. Using a pumping station example, the lesson demonstrates how reliability curves guide decision-making for series, parallel, and ‘𝑘’ out of ‘𝑛’ systems. It also explains key mathematical principles, such as squares and square roots, to calculate the required reliability levels for different system configurations. By analyzing these curves, engineers can optimize designs to balance cost, performance, and warranty requirements.
-
Lesson 11: Failure Rates and the MTTF / MTBFLesson 11 explores the concepts of Mean Time to Failure (MTTF) and Mean Time Between Failures (MTBF), highlighting common misconceptions that can lead to poor decision-making. The lesson explains probability density curves and how they relate to reliability curves. It also demonstrates how hazard rates vary based on different factors and failure mechanisms. It also contrasts early "wear-in" failures, typically caused by manufacturing defects or pre-existing damage, with "wear-out" failures due to accumulated damage over time. Ultimately, the lesson emphasizes that MTTF and MTBF alone do not accurately describe system reliability which depends on ‘how’ the item fails.
-
Lesson 12: Component and System MTBFsLesson 12 explores how component MTTFs and MTBFs relate to system MTTF and MTBF, along with several popular misconceptions about this relationship. While these metrics are often used to describe reliability, they do not fully capture the wear-in and wear-out failure mechanisms that affect real-world systems. The lesson highlights the importance of understanding time to failure distributions and system-level failure characteristics rather than relying on overly simplistic constant hazard rate assumptions. It also examines how component MTTFs and MTBFs relate to system-level reliability and why using these metrics in isolation can lead to misleading conclusions.
-
Lesson 13: Complex System Reliability AnalysisLesson 13 explores the reliability analysis of complex systems, focusing on a nuclear power plant’s secondary cooling loop. It demonstrates how fault tree models help break down system reliability using series and parallel configurations, leading to a 200-year reliability estimate. The lesson also introduces "rare event approximation" to simplify large-scale reliability calculations and highlights the importance of identifying critical components through "importance analysis." These techniques ensure reliability engineering efforts are targeted where they will have the greatest impact.
-
Lesson 14: Bridging Systems & Reliability Block DiagramsLesson 14 explores the limitations of FTA in modelling complex system configurations, particularly bridging systems that incorporate both series and parallel elements. By analyzing two four-pump system configurations, the lesson demonstrates how fault trees can model reliability through logical groupings of components. However, bridging systems introduce complexities that make fault trees challenging to use, leading to the introduction of Reliability Block Diagrams (RBDs) as an alternative. RBDs offer a more intuitive way to represent system reliability by focusing on success paths, making them particularly useful for modelling bridging systems.
-
Lesson 15: Other Logic Gates & ModelsLesson 15 explores advanced FTA concepts by introducing additional logic gates and their applications. The lesson examines systems with multiple instances of the same basic event and how this affects reliability calculations. The lesson also covers uncommon logic gates like XOR and NOT, demonstrating their relevance in modelling complex systems such as bridging systems. Through step-by-step analysis, it highlights how logic gate arrangements can simplify reliability calculations for intricate system configurations.
-
Lesson 16: Fault Trees & Cut SetsLesson 16 explores the concept of "cut sets" which are groups of component failures that lead to system failure. The lesson emphasizes "minimal cut sets," which contain the smallest number of failures required to cause a system breakdown, helping engineers identify single points of failure. Through examples like a nuclear power plant’s secondary cooling loop, the lesson illustrates how fault trees and RBDs can be used to find minimal cut sets. This analysis is crucial for improving system reliability, optimizing redundancy, and supporting decision-making in engineering design.
-
Lesson 17: Dependance“Dependence” refers to the interconnected nature of some component failures, where one failure may influence another. While assuming independence simplifies calculations, it introduces errors when common-cause failures (CCFs) exist. CCFs, such as manufacturing defects or shared environmental stressors, can significantly impact redundancy and overall system reliability. Understanding and modelling dependent failures—whether through common-cause events, switching systems, or load-sharing—helps engineers design more robust systems and avoid over- or under-estimating reliability.
-
Lesson 18: Advanced – Switching SystemsSwitching systems enhance reliability by delaying the wear and degradation of redundant components until they are needed. Unlike standard parallel systems, switching systems protect the standby component, reducing unnecessary damage and extending its usable life. However, analyzing switching system reliability is complex due to dependencies between components, as shown in fault trees and probability density curves. Simplified models, such as the "perfect switching system," often introduce inaccuracies that must be carefully considered in real-world reliability assessments.
-
Lesson 19: Advanced – Monte Carlo SimulationMonte Carlo Simulation uses repeated random sampling to analyze complex systems, generating a range of possible outcomes based on probability distributions. This method is particularly useful for reliability analysis, as it allows engineers to model failure scenarios that are difficult to solve analytically. By simulating component failures in a two-pump switching system, Monte Carlo techniques approximate system reliability curves, revealing key insights into failure patterns. The ability to generate thousands or even millions of data points enhance accuracy, making Monte Carlo Simulation a powerful tool in system reliability analysis.
-
Lesson 20: Fault Trees & RCALesson 20 dives into fault trees and RCA. RCA helps identify the fundamental reasons behind failures, ensuring effective corrective actions (CAs) to prevent recurrence. FTA plays a vital role in RCA by visually mapping failure pathways, but its purpose differs from reliability modelling. True root causes are actionable, meaning they stem from decisions or processes within an organization’s control. This lesson emphasizes the importance of a structured RCA approach, avoiding blame, and focusing on practical solutions to enhance system reliability.
-
Lesson 21: Smart Lock RCAThe lesson explores RCA using a smart lock failure as a case study, demonstrating how FTA can systematically identify failure mechanisms and root causes. It emphasizes the importance of analyzing failures layer by layer rather than jumping to conclusions, ensuring a thorough investigation. The lesson contrasts routine RCA with large-scale investigations like the Columbia Space Shuttle disaster, highlighting the balance between practicality and exhaustive analysis. Finally, it stresses the role of leadership in reliability engineering, reinforcing that identifying failures is only valuable if corrective actions are implemented.
-
Lesson 22: Smart Lock RCA Example - SoftwareLesson 22 explores RCA in software, using a smart lock as an example. Unlike hardware, software failures stem from coding errors, bugs, or defects that cause unexpected behaviour. The lesson examines common software failure modes, such as faulty state management and race conditions, and how they impact product reliability. By integrating structured RCA methods with tools like the Common Defect Enumeration (CDE) and fault trees, engineers can diagnose software failures and implement corrective actions or mitigations to enhance system performance.
-
Lesson 23: The Quality & Reliability MindsetA true “quality and reliability mindset” ensures that potential failures are addressed from the very first design phase, rather than being an afterthought. This approach integrates Failure Modes and Effects Analysis (FMEA) and Highly Accelerated Life Testing (HALT) early to identify and mitigate weak points before they become costly problems. By focusing on the ‘vital few’ failure modes, organizations can avoid expensive redesigns and shorten development timelines. Companies that embrace this mindset not only improve product reliability but also gain a competitive advantage by delivering higher-quality products with fewer production and warranty issues.
-
Lesson 24: Robust, Customer-centric DesignA robust, customer-centric design ensures products are both highly functional and free from predictable failure modes. By clearly defining requirements, functions, specifications, and failures, teams can create products that meet user needs while minimizing defects. FTA can help identify not just hardware and software failures but also usability and accessibility concerns that impact customer satisfaction. Prioritizing essential features and potential failure points from the start prevents costly redesigns and ensures products are both innovative and reliable.
-
Lesson 25: What Customers WantIn this lesson, we review how FTA helps engineers and users align their understanding of what constitutes a failure, ensuring system design meets real-world needs. In military applications, such as general service vehicles, failures range from minor inconveniences to mission-critical breakdowns. Defining and prioritizing failures through tailored severity scales prevents ambiguity and improves design guidance. By involving key stakeholders early, engineers can focus on addressing the failures that matter most, leading to more reliable and user-centered systems.
-
Lesson 26: The FTA Procedure – PreparationLesson 26 of the FTA Course focuses on the essential preparation steps required for a successful FTA workshop. It emphasizes the importance of defining the decision being analyzed, clearly identifying top events, describing the item under evaluation, and establishing the scope, limits, and resolution of the analysis. These foundational steps ensure that the subsequent brainstorming and analysis phases are efficient and effective, reducing wasted effort and aligning the FTA with decision-making objectives. Proper preparation, led by the FTA facilitator, is critical for generating meaningful and actionable insights.
-
Lesson 27: The FTA Procedure – ExecutionLesson 27 of the FTA Course focuses on the execution phase of the FTA process, detailing key steps such as team assembly, defining ground rules, brainstorming input events, and documenting rationale. Effective facilitation is emphasized, ensuring logical event analysis while maintaining engagement and structure. The lesson highlights the iterative nature of fault tree construction, culminating in analysis and interpretation to generate decision-actionable insights. Ultimately, the goal is to provide meaningful recommendations that aid decision-makers rather than simply showcasing the process.
-
Lesson 28: Fault Trees – Pros & ConsFTA is a powerful tool, but it is not universally applicable. This lesson explores the strengths and limitations of FTA compared to other system reliability modelling approaches, such as RBDs and event trees. While FTA excels in logical reasoning, root cause analysis, and incorporating various event types, it struggles with modelling physical system layouts, redundancy behaviours, and time-based failure sequences. Understanding the pros and cons of FTA ensures its appropriate use in system analysis and decision-making.
Instructor Information
Dr Jackson co-founded Acuitas in 2010 with a focus on quality and reliability engineering training.
Dr Jackson holds a Ph.D. and MSc in Reliability Engineering from the University of Maryland’s Center of Risk and Reliability. He also holds a BE in Mechanical Engineering, a Masters of Military Science from the Australian National University (ANU) and has substantial experience in the field of leadership, management, risk, reliability and maintainability. He is a Certified Reliability Engineer (CRE) through the American Society of Quality (ASQ) and a Chartered Professional Engineer (CPEng) through Engineers Australia.
Dr Jackson was the inaugural director of the University of California, Los Angeles (UCLA’s) Center of Reliability and Resilience Engineering and the founder of its Center for the Safety and Reliability of Autonomous Systems (SARAS). He has had an extensive career as a Senior Reliability Engineer in the Australian Defence Force, and is the Director of Acuitas Reliability Pty Ltd.
He has supported many complex and material systems develop their reliability performance and assisted in providing reliability management frameworks that support business outcomes (that is, make more money). He has been a reliability management consultant to many companies across industries ranging from medical devices to small satellites. He has also led initiatives in complementary fields such as systems engineering and performance-based management frameworks (PBMFs). He is the author of two reliability engineering books, co-author of another, and has authored several journal articles and conference papers.
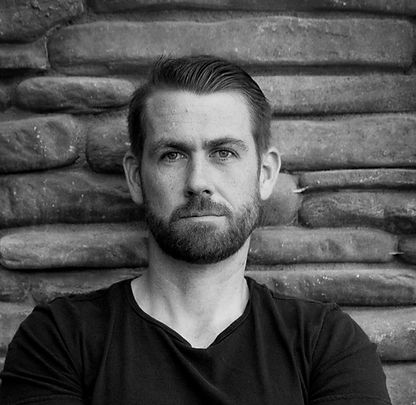
Need support in something else?
We offer services that range from helping you come up with a reliability plan for your organization or team through to helping you with a specific reliability engineering activity (like FMEA or HALT).
We can also help you with data analysis, organizational assessments, test planning, ALT, or any other reliability & quality training needs.
We can either come to you in person, or host an online course for your organization to improve your reliability engineering training.